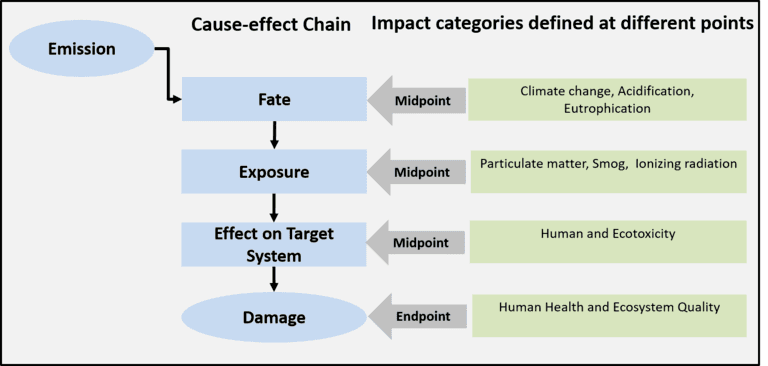
By Harnoor Dhaliwal and Pete Dunn
Corporations are increasingly applying Life Cycle Assessment (LCA) techniques in innovative ways. Once used primarily for internal evaluations of production processes, materials, and packaging, LCA is emerging as a useful tool for strategic assessments of sustainability efforts, risk management, and marketing communication.
This makes it increasingly important for executives to have at least some familiarity with how LCA works, so they can understand its potential and limitations – and for sustainability practitioners to be clear on how essential details impact major decisions. A good place to start is with Life Cycle Impact Assessment (LCIA), the point in the LCA process where potential environmental impacts of a product or service are identified and quantified.
Predicting these types of consequences can be complex, due to the long, interconnected pathways of interaction between environment and human activity, and to an outsider the process can seem mysterious and murky. But decades of development have resulted in a robust LCIA process capable of providing reliable insights into the impacts of materials and manufacturing processes. This article offers a concise overview of the steps and techniques involved, in a format that seeks to be both an accessible introduction for executive readers and a useful refresher for sustainability practitioners.
How LCIA addresses complexity
One of the basic challenges of LCIA is that the interaction pathways between ecosystems and pollutants are typically nonlinear and are affected by multiple variables. For example, the biological response of an organism might not increase in direct proportion to increases in a pollutant’s concentration – in many cases, once an inflection point is reached, the response can increase more rapidly.
Fortunately, we can draw on mathematical models to create a simplified version of reality that enables us to predict, with some amount of acknowledged uncertainty, the possible outcomes of an upstream decision. These models can mimic the natural processes the emission will go through -- and the impact it will have on human health and plant and animal species. Some of the commonly used models in LCA are USEtox for toxicity, the IPCC model for climate change and EUTREND for acidification.
In LCIA, impacts are modeled in three distinct phases: fate, exposure and effect, as shown in Exhibit 1 below.
- Fate modeling accounts for the characteristics of an emission and the environmental concentration it forms once released. This tells us where in the environment the emission ends up and its final concentration.
- Exposure modelling looks at the intake level of the emission by considering various routes and modes of intake. In other words, how much of the emission gets eaten, drunk, inhaled, absorbed, etc. For ecosystems, exposure models consider the amount of the emission that becomes bioavailable (i.e., able to be taken up by organisms).
- Once exposure is assessed, effect models link this information to known toxicity data at those intake levels. This allows us to assess the relative danger of exposure.
With this overview in mind, we can consider each of the three phases.
Using temporal and spatial integration to model fate
To model fate, we begin by calculating a steady-state environmental concentration of the pollutant. Before arriving at this steady-state, the pollutant generally goes through multiple environmental mechanisms, such as transport, degradation, volatilization, sedimentation or immobilization (exhibit 2).
The ultimate concentration is calculated by using steady-state box models where the concentration of the pollutant inside the box does not change, i.e., remains in a steady-state (exhibit 3). This figure shows a simplified version of a fate model; it calculates a steady-state concentration for a given volume, drawing on known pollutant inflow source rates and pollutant residence times.
The advantage of this type of model is that it allows each emission to be related to a single time-integrated fate value instead of multiple values at different points in time.
In addition to this temporal integration, fate models also use spatial integration – in other words, they do not detail where the emissions take place. Typically, the only spatial information included is the type of environmental media, such as air, surface water, sea water, soil, sediment, etc.
These temporal and spatial integrations are the two primary model simplifications that enable us to reduce complexity while still obtaining valid results. Newer models, however, are attempting to include more spatial details to reduce uncertainty levels, which can help make them more broadly applicable. For example, the IMPACT World+ method uses the spatially differentiated USEtox toxicity model that includes 17 sub-continental zones, such as Central America, North America, Europe, the Middle East and Southeast Asia.
Fate models also consider at least three emission compartments (air, water, and soil) and at least four transport compartments (air, water, soil, and sediment). The transport compartments account for multimedia transport, i.e., transport from one media to another, such as air to water (acid rain is one example). And since an emission can easily be distributed over multiple compartments, most emissions are linked to multiple fate factors.
Modelling Exposure: Intake and Bioavailability
Once fate is calculated, the next step is modeling exposure. An exposure factor for a pollutant links the fate factor to the intake level (for humans) or the amount that becomes bioavailable (for other organisms) via a specific environmental medium (air, water or soil).
Human exposure can occur through various routes, including inhalation, ingestion and absorption through skin (see exhibit 4 below). Modeling of exposure through water and soil can be more complex than exposure through air, simply because of the large variety of food and drink that humans consume. Generally, exposure to pollutants in water occurs through direct consumption of the water, or indirectly through consumption of seafood. For pollutants in soil, exposure can occur through food or direct ingestion or inhalation of dust.
To model exposure pathways as accurately as possible, current toxicity models include a wide range of foods including most grains, fruits, vegetables, dairy products, meat and fish.
The following expressions (Equation 1 and 2) can be used to calculate exposure for humans and ecosystems, and see the relationship between intake and concentration of emissions:
Toxicity data for modeling effects
We can, finally, estimate the relative danger of an emission by linking this exposure factor to adverse effects at a given exposure level, using an effect factor. Effect is often determined with toxicity data, using dose-response curves that represent the toxicological effects of a pollutant on a population at different doses.
For human toxicity, these data could be based on ED50 (the dose at which 50% of the individuals of a population are affected), while ecosystem analyses often use HC50 (the hazardous concentration at which 50% of species are affected). Note that the ED50 data are generally based on tests in rats, mice, hamsters and nonhuman primates. The effect factor for human toxicity is measured as cases per kg intake, while the effect factor for ecosystems is measured as the fraction of species exposed to concentration above their EC50 (Potentially Affected Fraction of Species, PAF per kg/m3).
The following expressions (Equation 3 and 4) can be used for calculating effect on humans and ecosystems:
Tying emission to impact: Characterization factors
We’ll close with a brief look at characterization factors that are used to convert an emission into an environmental impact. A characterization factor can be derived in the following manner:
Characterization factor (Q) = fate (F) x exposure (X) x effect (E)
Not all impact categories use all three factors. Toxicity impact does, but Smog and Particulate Matter impacts, for example, may use only fate and exposure, while Climate Change and Acidification impacts may use only fate. Please note that the characterization factors can also be defined at the damage level which is the endpoint of the cause-effect chain. The calculation of the damage factors generally involves the use of fate, exposure and effect factors.
For more information on impact assessment methods and models, please see the free online course on impact assessment and the free brown bag webinars offered by Earthshift Global.
Image credit: Pexels
Harnoor Dhaliwal is a certified LCA consultant at EarthShift Global. She holds a Bachelor’s degree in Botany from University of Delhi, India, and a Master’s degree in Environmental Policy Studies from New Jersey Institute of Technology. She did her graduate research work on sustainable remediation of contaminated sites. At EarthShift Global, Harnoor has carried out ISO-compliant Life Cycle Assessment studies on products including biofuels, packaging materials, food products, medical and pharmaceutical products, and industrial equipment. She has also developed and taught LCA courses. Her current focus is evaluating social Life Cycle Assessment and its application.
Pete Dunn, EarthShift Global’s marketing consultant, is an entrepreneurial marketing and communications strategist and writer, serving clients in academia, technology and B-to-B marketing. His journalism background includes eight years as founder, editor and publisher of WaferNews, the leading news publication for the international semiconductor manufacturing community. He specializes in creative collaboration and translating complex subjects into clear messages that inform and inspire.
TriplePundit has published articles from over 1000 contributors. If you'd like to be a guest author, please get in touch!